Controlled nuclear fusion has taken a solid step! my country’s "artificial sun"
The world's first fully high-temperature superconducting tokamak device has been built and put into operation in Shanghai, marking China's leading global completion of the engineering feasibility verification of high-temperature superconducting tokamaks.
01
"Honghuang 70" Achieves Plasma Discharge
Recently, Energy Singularity, a fusion energy commercial company based in Shanghai, announced that the Honghuang 70 device, designed, developed, and constructed by Energy Singularity, has successfully achieved plasma discharge. This is the world's first fully high-temperature superconducting tokamak device and also the first superconducting tokamak device developed and built by a commercial company.
The tokamak device, known as the "artificial sun," is widely used globally for the research and development of controllable nuclear fusion power generation. High-temperature superconducting tokamak devices are small in size and low in cost, possessing the potential for commercial power generation. The operation of this device signifies that China has been the first globally to complete the engineering feasibility verification of high-temperature superconducting tokamaks.
Starting with the construction and operation of "Honghuang 70," Energy Singularity plans to invest in the research and development of the next-generation strong magnetic field high-temperature superconducting tokamak device "Honghuang 170," which aims to achieve a deuterium-tritium equivalent energy gain (Q) greater than 10. The device is planned to be completed by 2027, with the goal of building a tokamak device suitable for a demonstration fusion power station after 2030.
Advertisement
Energy Singularity introduced that "Honghuang 70" has conducted discharge experiments based on two pre-ionization methods: local helicity magnetic flux injection (electron gun) and ion cyclotron resonance heating (ICRF), and successfully obtained the first plasma. "Honghuang 70" has a central magnetic field strength of 0.6 Tesla, a plasma major radius of 0.75 meters, and its magnet system is composed of 26 high-temperature superconducting magnets. This is the world's first fully high-temperature superconducting magnetic confinement fusion device and is also one of the four fully superconducting tokamaks in operation globally.A Solid Step Forward for Controllable Nuclear Fusion
As a crucial component of the new productive forces, controllable nuclear fusion is considered the "ultimate energy source for humanity," also known as the "artificial sun." It possesses numerous advantages such as being limitless, economical, plannable, clean, and safe, making it one of the important ways to address energy and environmental issues.
Nuclear energy is a new member of the energy family, which includes two main forms: fission energy and fusion energy. Fission energy is the significant energy released when the nuclei of heavy metal elements undergo fission. The development of controlled nuclear fission technology has made the application of fission energy commercially viable, such as in nuclear (fission) power stations.
Fission requires heavy metal elements like uranium, which are scarce on Earth, and conventional fission reactors produce radioactive nuclear waste, factors that limit the development of fission energy. Fusion energy is the energy released when two lighter atomic nuclei combine to form a heavier atomic nucleus. Current research on controlled nuclear fusion is dedicated to achieving the peaceful use of fusion energy.
There are two main types of research on controlled thermonuclear fusion: inertial confinement fusion and magnetic confinement fusion. The former uses ultra-high-intensity lasers to irradiate deuterium-tritium targets in an extremely short time to achieve fusion, while the latter takes advantage of the strong magnetic field's ability to effectively confine charged particles, containing deuterium-tritium gas in a special magnetic container and heating it to billions of degrees Celsius to achieve the fusion reaction.
The tokamak, invented by Soviet scientists in the 1950s, is a toroidal magnetic confinement device for controlled nuclear fusion experiments. After nearly half a century of effort, the scientific feasibility of generating fusion energy in a tokamak has been demonstrated, but the results are all produced in short pulses, which is quite different from the continuous operation of an actual reactor.
The successful application of superconducting technology to the coils that generate the strong magnetic fields in tokamaks is a significant breakthrough in the research of controlled thermonuclear fusion energy. Superconducting tokamaks enable the continuous and steady-state operation of magnetic confinement configurations, and they are recognized as the most effective way to explore and solve the engineering and physical problems of future fusion reactors.
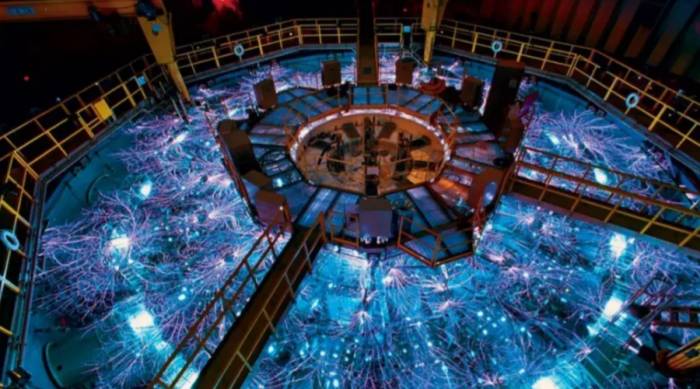
Tokamaks are essentially pulse devices because the plasma current is driven by induction. However, there is a possibility for so-called "advanced tokamak" operation, where they can achieve operation by combining non-inductive external driving and the natural pressure-driven current occurring within the plasma.The Institute of Plasma Physics primarily focuses on the research of high-temperature plasma physics, controlled thermonuclear fusion technology, and the development of related high technologies. It undertakes the construction and research tasks of the national nuclear fusion major science project, and has successively built Tokamak experimental devices such as HT-6B and HT-6M.
By the end of 1994, the Institute of Plasma Physics successfully constructed China's first large superconducting Tokamak device, HT-7, marking China's entry into the research phase of superconducting Tokamaks. The research achievements have attracted widespread attention from the international fusion community. The implementation of the "Ninth Five-Year" National Major Science Project—the large non-circular cross-section fully superconducting Tokamak nuclear fusion experimental device EAST plan—signifies China's entry into the experimental research phase of international large-scale fusion devices (near-core parameter conditions), indicating that China's nuclear fusion research has gained an important position on the international stage.
As the only magnetic confinement fusion technology route that has completed scientific feasibility verification, the Tokamak has always been the focal point of global controllable nuclear fusion research and development. Among them, the high-temperature superconducting Tokamak combines mature physics with engineering innovation, offering the potential to significantly enhance the cost-performance ratio of the device and accelerate the commercialization of fusion energy, becoming the fusion energy research direction that attracts the most market-oriented funding worldwide.
Steady Progress Towards the Ultimate Energy for Humanity
Humanity has now developed various new energy sources, such as solar, wind, hydro, tidal, and geothermal energy. These new energy sources each have their characteristics and practical value. However, fundamentally, they can only serve as supplementary energy sources. It is currently impossible for these energy sources to serve as the basic power source for the entire society's production and human life needs.
Nuclear power plants, which began to emerge on the world energy stage in the 1950s, have demonstrated tremendous power. However, these power plants are based on the fission reaction of atomic nuclei, and the disposal of radioactive waste generated is relatively difficult. Moreover, the reserves of the main nuclear fuel, uranium, are not abundant compared to other elements, and its mining and refining are very challenging. Additionally, due to some major accidents in its development history, people have lingering fears about nuclear power plants, and many countries have made decisions to no longer develop fission nuclear power plants. Therefore, fission energy from atomic nuclei is not the most ideal energy source for humanity.
Compared to other energy sources, the advantages of nuclear fusion are quite evident—1. Abundant raw material resources. The primary fuel, deuterium, combines with oxygen to form heavy water in the ocean, with each kilogram of seawater containing 0.03 grams of deuterium. There are 10^21 kilograms of seawater on Earth, containing 3 x 10^16 kilograms of deuterium. The current global energy consumption is approximately 2 x 10^20 joules per year, and burning just 10^6 kilograms of deuterium would suffice. The deuterium on Earth could last for 3 x 10^10 years, and even considering the annual increase in energy consumption levels, it would be sufficient for 10^10 years, which is hundreds of billions of years.
2. High energy release. The energy released per unit mass of fusion fuel is enormous, four times that of fission. "Burning" one kilogram of deuterium is equivalent to four kilograms of uranium, which is comparable to seven thousand tons of gasoline or ten thousand tons of coal. That is, "burning" 1 kilogram of seawater equals the energy obtained from burning 210 kilograms (300 liters) of gasoline.
3. Low radioactivity hazard. Helium produced in fusion reactions such as deuterium-tritium does not emit radioactivity. Unless fission materials like uranium or thorium are added to the fusion reactor, the radioactive waste generated is primarily leaked tritium, along with neutrons and protons released during fusion. The radioactivity from a fusion reactor is significantly less than that from a fission reactor.
4. High safety. Fusion is a self-limiting process—if the reaction becomes uncontrollable, it will stop on its own. Since fusion reactions can only occur under extreme conditions, chain reaction-based fission accidents or nuclear meltdowns are impossible.
However, achieving nuclear fusion requires an initial input of energy to help atomic nuclei overcome electrostatic repulsion. The fundamental reason why controlled fusion research is so challenging is that all atomic nuclei carry a positive charge, and as two positively charged nuclei approach each other, the electrostatic repulsion between them increases.
Nuclear force is a short-range force, and they must be brought close enough, within 10^-15 meters, for the force to "bind" them into a whole, forming a new atomic nucleus. Fission of uranium-235, plutonium-239, etc., does not require any kinetic energy from the incoming neutron or the target atomic nucleus; whereas for two atomic nuclei to fuse, one or both must first have sufficient energy to overcome the electrostatic repulsion between them.
The sun is constantly undergoing nuclear fusion reactions, with a core temperature of 15 million degrees and a surface temperature reaching up to 6000 degrees. However, due to the immense pressure, fusion reactions occur naturally, but the conditions required for sustained nuclear fusion on Earth are much more stringent than those on the sun.
Ultra-high temperature is an essential external condition for nuclear fusion, helping atomic nuclei gain energy. The most convenient way to obtain fusion energy is to heat a large amount of fusion material to an extremely high temperature in a very short time. The higher the temperature, the faster the deuterium nuclei move. When the temperature reaches 100 to 200 million degrees Celsius, the speed of deuterium nuclei movement also reaches 1000 to 2000 kilometers per second.
According to an article on the official website of the Institute of Plasma Physics, Chinese Academy of Sciences, based on experimental data, for two deuterium nuclei to meet, their relative speed must be greater than 1000 kilometers per second, which means the temperature must be as high as 100 million degrees Celsius. Therefore, ultra-high temperature is a necessary external condition for nuclear fusion to occur. Using conventional methods, it is very difficult to heat to such high temperatures. After the successful development of the atomic bomb in 1945, people also found a way to heat to several hundred million degrees in an extremely short time.However, "fusion ignition" is just the first step; engineering applications also require the achievement of net fusion energy. Utilizing the energy released from the fusion reaction to maintain extremely high temperatures, without the need for further external energy input, the reaction can sustain itself indefinitely, at which point the "burning" of fusion fuel in the "reactor" has been ignited.
The scientific term that characterizes this concept is "fusion ignition." For a certain temperature, the number of collisions between atomic nuclei within a certain time is proportional to the density of atomic nuclei in the plasma; and at a certain density, the number of collisions between atomic nuclei is proportional to the time (confinement time) that the plasma maintains this density. Therefore, the release of energy in the fusion reaction is related to the product of the plasma's temperature, atomic nucleus density, and confinement time (the fusion triple product).
British scientist Lawson detailed the necessary conditions for fusion ignition in the 1950s, and thus the ignition conditions are also known as the Lawson criterion. According to the Lawson criterion, only when the fusion triple product exceeds a certain value can an effective fusion power output be produced.
Achieving "ignition" is only the first step in controlled nuclear fusion research. The second goal is to make the output energy exceed the input energy, obtaining net fusion energy. Scientists refer to the first goal as verifying scientific feasibility and the second goal as verifying engineering technology feasibility.
The ultimate goal of all tokamaks is to heat the deuterium-tritium fusion fuel to the ignition point or higher temperatures and to control and sustain the reaction for as long as possible to pursue continuous fusion energy output.
The magnetic system in a tokamak is an important part of generating and controlling the magnetic field. The required magnetic field strength for operation is extremely high, and large currents must be passed through the magnetic coils. Conventional metal conductors suffer from significant resistive losses. Even using copper, which has good conductivity, to wind the coils, the inevitable heat generation due to the large currents limits the long-term steady-state operation of magnetic confinement nuclear fusion.
Due to the zero-resistance effect of superconductors and their ability to carry higher current densities, which is beneficial for constructing more compact, higher-field-strength fusion devices, they can effectively improve long-pulse steady-state operation. In the late twentieth century, scientists began to apply superconducting technology to tokamak devices.
With significant progress in many large and medium-sized tokamaks worldwide, in 1985, the Soviet Union and the United States proposed at the Geneva Summit to jointly initiate the "International Thermonuclear Experimental Reactor (ITER)" project. In February 2003, China officially joined the ITER project negotiations. In November 2006, China signed the "Agreement on the Joint Implementation of the International Thermonuclear Experimental Reactor Project to Establish the International Fusion Energy Organization" in Paris, France, along with representatives from the European Union, India, South Korea, Russia, and the United States.The ITER project aims to construct a nuclear fusion experimental reactor, verifying the scientific and technological feasibility of the peaceful use of nuclear fusion energy. The ITER plan is the first large-scale international scientific and technological project that our country has participated in with full equality, and it is also the largest international scientific and technological cooperation plan that we are currently participating in as a full-fledged partner. As one of the participants, our country will undertake the "procurement package" tasks in five major areas, including superconducting materials, power supplies, blanketing, remote sensing technology, and fueling systems.
Fusion is an important part of our country's nuclear energy strategy of "three steps." The research on nuclear fusion energy in our country began in the early 1960s. Despite facing a very difficult environment for a long time, it has always been able to maintain a stable and gradual development. From the very beginning, the research on nuclear fusion in China has aimed primarily at achieving controlled thermonuclear fusion energy within our country.
Starting from the 1970s, the tokamak was chosen as the main research path, and small-scale devices such as CT-6 (Institute of Physics, Chinese Academy of Sciences), KT-5 (University of Science and Technology of China), HT-6B (Institute of Plasma Physics, Chinese Academy of Sciences), HL-1 (Southwest Institute of Physics, Nuclear Industry), and HT-6M (Institute of Plasma Physics, Chinese Academy of Sciences) were successively built and operated. In 1983, our country proposed the "thermal reactor-fast reactor-fusion reactor" nuclear energy development strategy of "three steps," which is of significant strategic importance.
Since the 1990s, our country has carried out a medium-sized tokamak development plan, exploring advanced tokamak economic operation models and issues related to the steady-state operation of tokamaks. In October 1990, after carefully analyzing the trends in international nuclear fusion development, the Institute of Plasma Physics of the Chinese Academy of Sciences formally reached an agreement with the Russian side to introduce the T-7 through barter trade.
Afterward, the Institute of Plasma Physics spent three years upgrading the T-7 device to the HT-7 device (Hefei Super Ring), especially reducing the number of toroidal field magnets to gain more space for plasma diagnostics, which is conducive to the conduct of physical experiments.
From its commissioning in 1994 to the final round of experiments in 2012, the success of HT-7 has allowed our country to accumulate rich experience in the experimental operation of superconducting tokamaks. However, the HT-7 device only used superconducting materials for the toroidal field magnets, while the central solenoid magnets used to excite the plasma and the poloidal field magnets used to control the plasma still used copper conductors.
In the future, fusion reactors need to develop towards the direction of steady-state nuclear fusion energy, and the all-superconducting tokamak is the foundation for steady-state operation. In 1996, the Institute of Plasma Physics proposed the construction plan for HT-7U to the state, and in October 2003, the device was officially renamed EAST, which is an acronym composed of the first letters of the words "Experimental," "Advanced," "Superconducting," and "Tokamak." The EAST device was built in 2006. The EAST device is the world's first all-superconducting tokamak device independently designed and developed by our country. Its successful construction and physical experiments have brought China to the forefront of the field of magnetic confinement fusion research, making China one of the important fusion research centers in the world.Nuclear fission opens the door to the utilization of nuclear energy
The fourth generation of nuclear power plants is drawing near
The practical significance of nuclear fission research for the general public is often reflected in nuclear power generation.
Since the first use of nuclear energy for electricity generation by the Experimental Breeder Reactor I (EBR-I) in the United States in December 1951, nuclear power plant technology can be broadly divided into four generations. The first generation pertains to prototype nuclear power plants, primarily aimed at demonstrating the feasibility of nuclear power in engineering implementation through experimental demonstration; the second generation mainly achieved commercialization, standardization, serialization, and mass production to enhance economic viability; the third generation varies by country, with representatives including the American AP1000, the French EPR, and China's Hualong One; the concept of the fourth generation nuclear energy system (distinct from nuclear power technology or advanced reactors) was proposed by the United States in the late 1990s, followed by the establishment of the "Generation IV International Forum (GIF)" by countries such as the United States, France, Japan, and the United Kingdom. The GIF's overall goal is to introduce a fourth-generation nuclear energy system (Gen-IV) to the market by around 2030 that can address the issues of nuclear energy's economic viability, safety, waste management, and non-proliferation.
The current mainstream third-generation technology is an inheritance and improvement based on the second-generation technology. The concept of third-generation nuclear power originated in the 1990s when the Electric Power Research Institute in the United States issued the "Advanced Light Water Reactor Utility Requirements" document (URD), which used a series of quantitative indicators to regulate the safety and economic performance of nuclear power plants. Subsequently, the European Utility Requirements for Light Water Reactors (EUR), issued by Europe, also expressed views that are the same or similar to the URD. Internationally, nuclear power units that meet the requirements of the URD or EUR documents are commonly referred to as third-generation nuclear power units.
Representatives of the reactor types of third-generation nuclear power plants include the AP1000 by Westinghouse Electric Company of the United States, the EPR by Areva of France, the AES-2006 by the Russian Atomic Energy Corporation, and China's "Hualong One" and "Guohuo One," among others.
The fourth-generation nuclear power represents a fundamental and revolutionary change, completely different from the third-generation plans and concepts. Whether it is the second or third generation, they all belong to thermal reactor technology, with the majority of reactor types being pressurized water reactors. Compared to the second generation, the third-generation technology does not have a substantial difference; it only offers a slightly improved safety level. The fourth-generation nuclear power technology is not an extension of the third-generation technology; it represents a fundamental and revolutionary change compared to contemporary nuclear power technology, including completely different plans and concepts.
The fourth-generation nuclear reactors are the next generation of reactors currently under development, featuring significant innovations in reactor concepts and fuel cycles. Their main characteristics include high safety and reliability, minimal waste production, better economic performance, multi-purpose functionality, and the ability to prevent nuclear proliferation.Through joint efforts, in 2002, the Generation IV International Forum (GIF) selected six nuclear energy systems from over 130 conceptual designs as the most promising fourth-generation nuclear energy technologies. These include the Gas-Cooled Fast Reactor (GFR), Lead-Cooled Fast Reactor (LFR), Molten Salt Reactor (MSR), Sodium-Cooled Fast Reactor (SFR), Supercritical Water-Cooled Reactor (SCWR), and Very High-Temperature Reactor (VHTR). Subsequently, a technology roadmap was published (updated in 2014), which identified and planned the essential research and development work and related timelines for the advancement of these six nuclear energy systems.
China began researching high-temperature gas-cooled reactors in the 1970s, with the main implementing body being the Institute of Nuclear and New Energy Technology at Tsinghua University. In 1986, the high-temperature gas-cooled reactor was listed as one of the national "863 Program" projects. With the support of the "863 Program," the first batch of concrete for the HTR-10 was poured in June 1995, it achieved criticality in 2000, and full-power commissioning was realized in January 2003, becoming the world's first pebble-bed modular high-temperature gas-cooled experimental reactor. In 2006, the high-temperature gas-cooled reactor was included in the national list of 16 major science and technology projects, officially putting its commercialization on the agenda.
China Huaneng Group, China National Nuclear Corporation, and Tsinghua University jointly invested in the construction of a 200 MW modular high-temperature gas-cooled reactor nuclear power plant demonstration project, with investment ratios of 47.5%, 32.5%, and 20%, respectively. The project was launched in October 2008, originally scheduled to start in September 2009, and to begin power generation in November 2013. However, due to the impact of the Fukushima nuclear accident, the official construction commencement was delayed until December 2012. The first fuel loading occurred on August 20, 2021, and the initial full-power operation of the dual-reactor system was achieved by the end of 2022. Currently, the project is undergoing the latest experimental validation, and as of June 30, 2023, it has not yet commenced commercial operation. Building on this, the technical research and development for China's 600 MW modular high-temperature gas-cooled reactor are also being planned.
With the successful construction of domestic HTR-10 and the Shi Dao Bay high-temperature gas-cooled reactor demonstration project, China's high-temperature gas-cooled reactor technology has achieved global leadership. The successful demonstration at the Shi Dao Bay nuclear power plant has laid the foundation for large-scale promotion. High-quality process heat and high-parameter high-temperature steam can be widely applied in the oil, chemical, and other industries. In April 2015, according to China National Nuclear Corporation, the preliminary feasibility study report for the commercial 600 MW high-temperature reactor project in Ruijin, Jiangxi, has passed expert review.
This is an important step for China National Nuclear Corporation on the path to commercializing the 600 MW high-temperature reactor, laying a solid foundation for the smooth progress of China's first commercial high-temperature reactor power station project. After obtaining national approval and a construction license issued by the National Nuclear Safety Administration, it is expected to take 4-5 years from the start of the first phase of the Ruijin high-temperature reactor nuclear power project to the grid connection of two units. In addition, with the support of the national science and technology major project "High-Temperature Gas-Cooled Reactor Nuclear Power Station," research on the key technology of hydrogen production by high-temperature gas-cooled reactors has also made good progress.
Overall, China's technological leadership in the field of fourth-generation nuclear power stations, mastery of independent intellectual property rights, and active international cooperation have given it significant influence in the global nuclear energy market. This also lays a solid foundation for the advancement of controlled nuclear fusion technology.
Comment